Processing, Printing, and the Lab-to-Fab gap for OPV: An Interview With ARMOR.
#TrendingBrilliant Matters interviews ARMOR experts on the challenges of industrial-scale printing of organic solar cells!
Among renewable sources of energy, solar is by far the most abundant and can provide our species with a virtually limitless source of energy. Solar cells thus have the potential to greatly accelerate our transition to 100 % renewable energy, given that we find innovative ways to mass produce them and deploy them quickly while limiting their usage on useful land. Organic PhotoVoltaic (OPV) cells are part of the new generation of thin-film photovoltaic technologies facing these challenges head-on and gearing up for mass production. These devices can be lighter, more flexible and easily integrated anywhere, including in smart cities with energy-producing solar windows, roofs, and walls. They could even revolutionize agrivoltaics by providing greenhouse solar cells that are transparent to the specific photon wavelengths plants use.
OPV technology is enabled by light-harvesting organic small molecules and polymers which can be dissolved into inks and then printed using roll-to-roll (R2R) equipment in essentially the same way we manufacture newspapers today! The research on OPV has evolved quickly in the past five years, with new organic semiconducting materials leading to almost a two-fold increase in performance. OPV have now breached the 18% Power Conversion Efficiency (PCE) mark in academic labs,1,2 a value close to commercially available silicon solar cells. With these advances, we now ask ourselves: Is it time to start thinking about mass production? Our answer is a resounding yes! However, there is still a big gap between lab cells and commercial OPV modules, which are currently three to four times less efficient. The chart in Figure 1 clearly shows the difference between lab-scale devices and commercial modules .
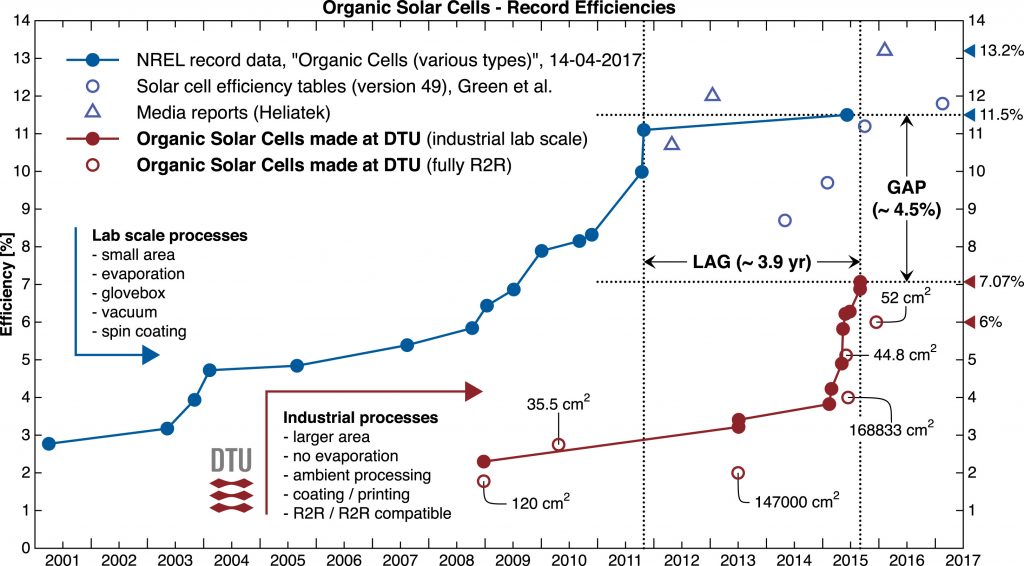
Figure 1: Chart Showing the Progress in Certified Efficiency for Organic Laboratory Solar Cells over Time vs. Scaled Data from the Technical University of Denmark. This data clearly shows the “scaling lag” between lab and fab for OPV cells. (Source: Jon E. Carlé, Martin Helgesen, Ole Hagemann, Markus Hösel, Ilona M. Heckler, Eva Bundgaard, Suren A. Gevorgyan, Roar R. Søndergaard, Mikkel Jørgensen, Rafael García-Valverde, Samir Chaouki-Almagro, José A. Villarejo, Frederik C. Krebs, Overcoming the Scaling Lag for Polymer Solar Cells, Joule, 2017, 1(2), 274-289.)
Which begs the question, why is the gap between lab and fab so large? To begin answering this question, we had a chance to talk to the experts at ARMOR about some of the challenges they face when adapting this new generation of high-performance OPV materials for their roll-to-roll production line. For those unfamiliar with ARMOR, they are currently one of the companies pioneering the transition of OPV to the commercial-scale printing press. Based in France, they have an ongoing project called ASCA® , which aims to use their world-leading expertise in R2R printing to bring to market a new generation of flexible OPV films.
Let us first introduce our experts from ARMOR and then hear from them on why R2R is the preferred method for large scale production of OPV:
Mélanie Bertrand
OPV Research, Development and Innovation Manager, ARMOR SA
Holding an engineering degree in materials and polymers sciences (ECPM Strasbourg), Melanie started her career as engineer in additive printing development. She has 6 years of experience in the formulation and industrialization of inks in the field of industrial inkjet printing. Looking for new challenges, she joined ARMOR in 2013 as Head of Materials and Formulations for OPV. Since 2016, she is also overseeing the encapsulation and characterization teams of the OPV activity, and grew into the role of OPV Research, Development and Innovation manager.
Damien Hau
OPV Research, Development, Innovation and Industrial Manager, ARMOR SA
Holding an engineering degree in chemistry and polymers sciences (Lille, France), Damien started in Research and development in Lafarge (France) (mineral chemistry) before he moved to BASF (Germany) in coating application development. Then he moved in Pyral (France) on the close development area as Laboratory manager and followed with his career in Bucarest (Romania) where he did install a plant of industrial paint and launch the commercial activity. Finally he joined Armor in 2006 to take the head of technical teams dedicated to OPV technology. From chemistry to production with some focus on process in Roll to Roll.
BM: Why did ASCA® choose roll-to-roll printing to produce organic solar cells?
Damien: ABL chose roll-to-roll for its low-cost production potential at large scale. Indeed, the fastest R2R coating equipment can run at over 1000 m²/ min. Even with the speed set at 50 m2/min we estimate that the process cost will come in at a cost of under 0,3 €/m². In addition, R2R processes using liquid inks permits the use of a wide range of raw materials, as we can optimize the ink formulation in front of such processes.
Wow that is fast! That means that this type of equipment could potentially print enough solar cells to cover a football field in about 10 minutes. Compared to what we are accustomed to in the R&D lab, for example spin-coating on a 1×1 cm substrate, this is orders of magnitudes larger in scale! In the same amount of time a researcher can make a small test solar cell, these printers can produce a mindboggling 109-1010 times larger area of solar cells. This also means, and this is important, that we can imagine ASCA would one day be able to produce more solar cell area than the largest solar cell manufacturer today, with just one printer.
BM: We have seen a lot of recent performance improvements in OPV, mainly linked to the introduction of new organic semiconductors. What are the most challenging aspects of transferring a new material from R&D to large-scale printing of OPV?
Damien: The most challenging step when transferring a material from lab to R2R is to find an appropriate process and ink formulation that can duplicate as close as possible the lab conditions. The second issue we could often see is that the scale-up of each component is not robust enough, giving some variation in the material’s properties that could be critical for the product.
For researchers in the field, we think it would be useful to know what type of criteria are important for the transfer of promising new materials to R2R printing. Could you tell us a bit more about how you evaluate these materials to determine their transferability to large scale printing techniques?
Mélanie: We use a multi-step protocol: First, the selection of materials is done with partners, with evaluation of the potential in academic conditions (controlled atmosphere, halogenated solvents, spin coating with evaporated top layers). Secondly, If the targeted performances are achieved, and the material is soluble in non chlorinated solvents, we switch to doctor blading processes, which are closer to roll-to-roll processes, and will evaluate the material in fully printed solar cells. Last but not least, we will transfer from lab cells (1cm², coated on glass) to our roll-to-roll R&D line, which is a miniature reproduction of our production line.
There are a lot of things to consider! Could you elaborate on what are the most important processing characteristics required for an organic semiconducting material to be used in a printed solar cell made by ARMOR?
Mélanie: There are a lot! Just to mention the most important ones: Semiconductors need to be soluble in non-chlorinated, non-toxic solvents. Drying and annealing are restricted as well, as it is not possible on roll to roll lines to include annealing steps for too long at temperatures >140°C. Then, morphology additives that stay in the coated film at these temperatures are not welcome. We need to respect our process windows, meaning that we must optimize the ink formulation concentration and viscosity to the targeted film thickness: not always so easy! To ensure a good batch to batch reproducibility is essential, not to have to adapt our formulations and process at each material reception Material stability (thermal and light stability) is also essential, and unfortunately not often assessed at academic development step. Air stability is a key point as well, as during production both powders and inks are processed in air.
BM: What other information are you looking for in research articles to evaluate the potential of new materials for large scale production?
Mélanie: Stability data in conditions close to real life of PV panels!
It may seem like the set of criteria outlined by our industry experts is quite strict, but in fact these are the essential criteria for creating efficient, long lasting, and environmentally friendly OPV modules at the industrial scale. Let us go over each of the points mentioned in more detail.
Processability:
A homogenous coating of the active layer is one of the most important factors we consider when trying to create high performance OPV cells. In the lab, researchers often rely on halogenated solvents, the best solvents around to solubilize organic semiconductors. As our industry experts pointed out, halogenated and highly toxic solvents are not welcome. In fact, they are massive health and safety hazards in a production context due to their toxicity and carcinogenic nature.3 Spin-coating techniques are also used because they are simple and efficient at small scale to produce high quality films on rigid substrates. This technique is not practical to produce large scale modules quickly and, as you might guess, rigid substrates are not an option for R2R equipment! So, can’t we just change the substrate and processing solvent and start printing? – Not exactly!
Sometimes, the best materials from the lab just won’t be compatible with the requirements laid out by industry. Adjustments often need to be made for these materials so that they can still form good quality films when processed from non-halogenated solvents onto flexible substrates. For instance, the solubilizing alkyl chains of polymers are lengthened, or additional monomers are strategically introduced which helps the material dissolve in non-halogenated solvents, while maintaining critical optical, electronic, and film-forming properties.
A great example of this strategy in action can be found in a recent report.4 The Y6 electron acceptor molecule, the current state-of-the-art in 2020, can give 15%+ PCE when spin-coated from chloroform with an appropriate donor material. When the solvent is changed for THF, however; the obtained PCE was almost cut in half to ~8% due to the poor processability of Y6 in non-halogenated solvents! To address this issue, a new variant of Y6 was created, where the 2-ethylhexyl side chains are replaced by 2-butyloctyl chains, dramatically increasing the processability of the material in nonhalogenated solvents such as THF. Optimized solar cells with PCE ~ 15 % could be obtained using this new derivative, whether by spin-coating or blade coating methods! This is certainly exciting news for anyone looking to scale up printing of cells using this material!
Do you have pictures of different devices with active layers showing good or poor processability?
Both: Yes, we do! here are some examples showing the possible impact on coating quality of a non optimized solvent / drying compared to a homogeneous coating:
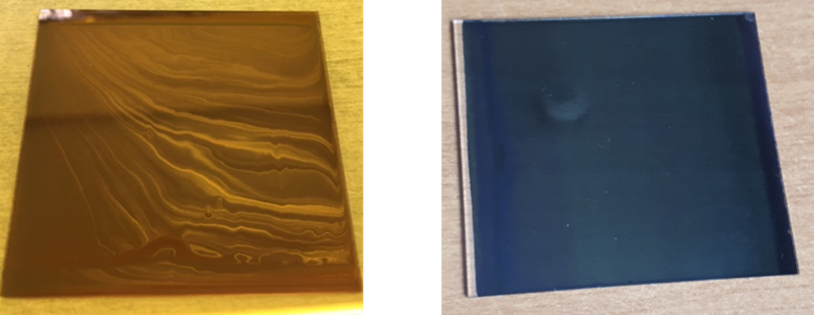
Figure 2: Left: example of a poor coating of organic semiconductor resulting from poorly optimized solvent/ drying conditions. Right. Example of a good, homogenous coating.
Stability:
Imagine spending the time to cover a whole skyscraper in OPV modules, only to have to replace them in a matter of a few months because they went bad! This would be time consuming and frustrating for the building owner, and probably not worth it in the long run. For this reason, it is important that the organic materials in the active layer have a high degree of stability.
“Stability” is a broad term which encompasses two general areas: materials stability and morphological stability. These aspects are just as important as the PCE of a solar cell but often they take a back seat! For instance, during the rapid development of the field, “non-fullerene acceptors” (NFA’s) emerged, circumventing the poor visible light absorption by fullerene derivatives, which led to dramatic increases in PCE compared to fullerene-based cells. Early work suggested that these new NFAs might even posses a higher degree of inherent photostability compared to their fullerene counterparts,5 but recent studies show that this might not always be the case.6 Fortunately, it is possible to rely on our old bag of chemistry tricks and molecular design to find new derivatives which do possess the desired stability under light. One study published in Joule in 2019 by Du et al. highlighted this strategy, demonstrating that simply changing methyl groups for fluorine atoms can have huge impact on the material’s stability towards light soaking (Figure 3). In the end they found that stable and efficient solar cells can be made using the fluorinated version of this material which could last up to 10 years while retaining 80%+ efficiency!
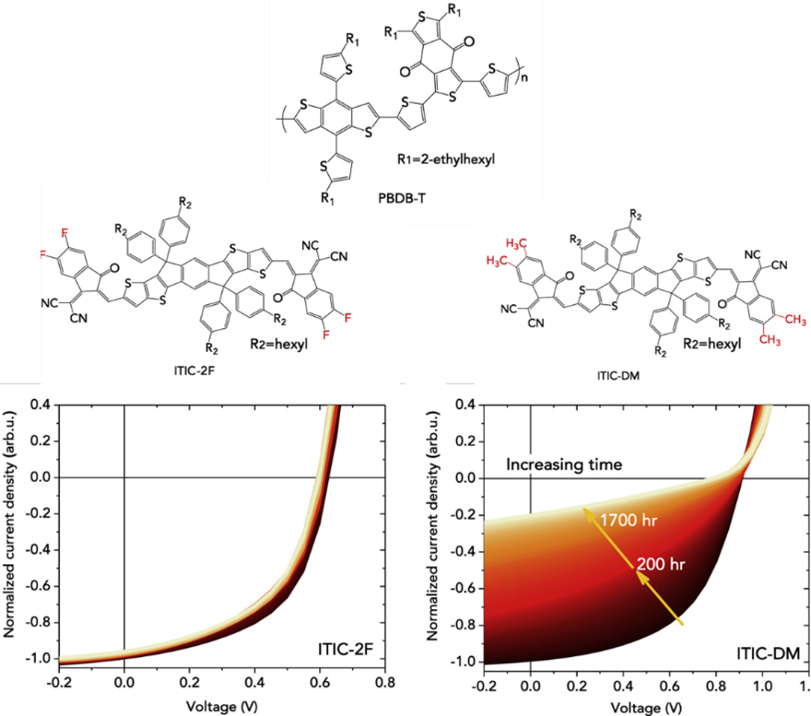
Figure 3: top: Chemical structures of PBDB-T, ITIC-2F and ITIC-DM. bottom: J-V curves from solar cells made using PBDB-T(donor) and either ITIC-2F or ITIC-DM (acceptors). Image from Du et al. Joule 3, 215–226, January 16, 2019. The solar cells were held under constant illumination in a nitrogen atmosphere.
In addition to photostability, It is also important that the materials are stable when heated to moderate temperatures (< 140 °C), are exposed to oxygen, or are in the presence of other components in the cell such as interlayers or additives. While encapsulation can help to avoid contact with oxygen, other chemical interactions are less avoidable. For example, one popular high performance NFA, ITIC, is now known to be unstable in basic conditions, such as when it is in contact with the common interlayer Polyethyleneimine (PEI).7 Thus, careful consideration of the chemical nature of each part of the solar cell is the key to resolving this issue.
How about morphological stability then? Have you ever shaken a bottle of salad dressing to mix it up and a few hours later it is totally separated into layers again? Well, since the active layer of an OPV cell is a heterogenous mixture of two or more components (like a salad dressing) the same thing (phase separation) can happen, and it is not good for performance! This is because the morphology of the active layer that gives the best performance is not usually the thermodynamically most stable one.8 Thus, over time (and with temperature) the molecules and polymers tend to rearrange inside the active layer and in turn performance is reduced. This is still a big challenge in the field and major work is being done in understanding the fundamental design principles for molecules which lead to both a stable morphology and a high PCE.
Batch Reproducibility:
Even a small variation in the materials properties can cause large differences in the printed films. Therefore, it is essential that each batch of active layer material to be printed has essentially the same properties. If properties such as solubility or viscosity are significantly different, then the whole production line needs to be modified to achieve the desired result! Batch-to-batch reproducibility can be especially problematic for polymeric materials which have inherent polydispersity and so no two batches are exactly the same. It is therefore important to have a robust synthetic method that ensures an accurate reproduction of the material. For a company like Brilliant Matters, this is an area of high priority to say the least!
Since the material designs and device efficiencies have rapidly evolved over the years, it is reasonable to anticipate more improvements as future generations of OPV materials are developed. Our last question: Do you think the new generation of organic semiconducting materials will bring new challenges in printed solar cells?
Damien: I can suppose that new semiconducting material will need new process conditions that don’t exist with current R2R equipment. These new materials will impose industry to re innovate their actual knowledge in R2R process.
This is a good reminder that even as OPV modules are on the verge of mass commercialization, we certainly remain at an exciting stage of rapid development, and we will surely have to keep our eye out for both new materials design and new device architecture!
In short, to make mass produced OPV using R2R equipment a reality, there are key features that must be found in the active layer materials. Processability from non-halogenated and non-toxic solvents is critical as large volumes of solvent will be used to print films at high speed on flexible substrates. Stability, both photochemical and morphological, is also a concern that is all too often ignored during new material discovery, but is so crucial for real-world application. Finally, a robust synthesis is required so that the material’s properties are accurately reproduced in each new batch. By following these general guidelines, researchers can really help to accelerate the realization of fully printed, high performance, mass produced OPV.
Learn more about Asca, OPV films by Armor on their website: https://www.asca.com/
Brilliant Matters is committed to being a part of the solution for industrially printed organic solar cells. To learn more about some of the materials that have the required features to be used in a printing process, consult our product pages:
References:
(1) Qishi Liu; Yufan Jiang; Ke Jin; Jianqiang Qin; Jingui Xu; Wenting Li; Ji Xiong; Jinfeng Liu; Zuo Xiao; Kuan Sun; Shangfeng Yang; Xiaotao Zhang; Liming Ding. 18% Efficiency Organic Solar Cells. Sci. Bull. 2020,65 (4), 272–275. https://doi.org/10.1016/j.scib.2020.01.001.
(2) Lin, Y.; Firdaus, Y.; Isikgor, F. H.; Nugraha, M. I.; Yengel, E.; Harrison, G. T.; Hallani, R.; El-Labban, A.; Faber, H.; Ma, C.; Zheng, X.; Subbiah, A.; Howells, C. T.; Bakr, O. M.; McCulloch, I.; Wolf, S. D.; Tsetseris, L.; Anthopoulos, T. D. Self-Assembled Monolayer Enables Hole Transport Layer-Free Organic Solar Cells with 18% Efficiency and Improved Operational Stability. ACS Energy Lett. 2020, 5 (9), 2935–2944. https://doi.org/10.1021/acsenergylett.0c01421.
(3) Wadsworth, A.; Ashraf, R. S.; Abdelsamie, M.; Pont, S.; Little, M.; Moser, M.; Hamid, Z.; Neophytou, M.; Zhang, W.; Amassian, A.; Durrant, J. R.; Baran, D.; McCulloch, I. Highly Efficient and Reproducible Nonfullerene Solar Cells from Hydrocarbon Solvents. ACS Energy Lett. 2017, 2 (7), 1494–1500. https://doi.org/10.1021/acsenergylett.7b00390.
(4) Hong, L.; Yao, H.; Wu, Z.; Cui, Y.; Zhang, T.; Xu, Y.; Yu, R.; Liao, Q.; Gao, B.; Xian, K.; Woo, H. Y.; Ge, Z.; Hou, J. Eco-Compatible Solvent-Processed Organic Photovoltaic Cells with Over 16% Efficiency. Adv. Mater. 2019, 31 (39), 1903441. https://doi.org/10.1002/adma.201903441.
(5) Zhang, G.; Zhao, J.; Chow, P. C. Y.; Jiang, K.; Zhang, J.; Zhu, Z.; Zhang, J.; Huang, F.; Yan, H. Nonfullerene Acceptor Molecules for Bulk Heterojunction Organic Solar Cells. Chem. Rev. 2018, 118 (7), 3447–3507. https://doi.org/10.1021/acs.chemrev.7b00535.
(6) Doumon, N. Y.; Dryzhov, M. V.; Houard, F. V.; Le Corre, V. M.; Rahimi Chatri, A.; Christodoulis, P.; Koster, L. J. A. Photostability of Fullerene and Non-Fullerene Polymer Solar Cells: The Role of the Acceptor. ACS Appl. Mater. Interfaces 2019, 11 (8), 8310–8318. https://doi.org/10.1021/acsami.8b20493.
(7) Hu, L.; Liu, Y.; Mao, L.; Xiong, S.; Sun, L.; Zhao, N.; Qin, F.; Jiang, Y.; Zhou, Y. Chemical Reaction between an ITIC Electron Acceptor and an Amine-Containing Interfacial Layer in Non-Fullerene Solar Cells. J. Mater. Chem. A 2018, 6 (5), 2273–2278. https://doi.org/10.1039/C7TA10306A.
(8) Ghasemi, M.; Hu, H.; Peng, Z.; Rech, J. J.; Angunawela, I.; Carpenter, J. H.; Stuard, S. J.; Wadsworth, A.; McCulloch, I.; You, W.; Ade, H. Delineation of Thermodynamic and Kinetic Factors That Control Stability in Non-Fullerene Organic Solar Cells. Joule 2019, 3 (5), 1328–1348. https://doi.org/10.1016/j.joule.2019.03.020.